New Study Illuminates Invisible Architects of the Ocean’s Carbon Cycle
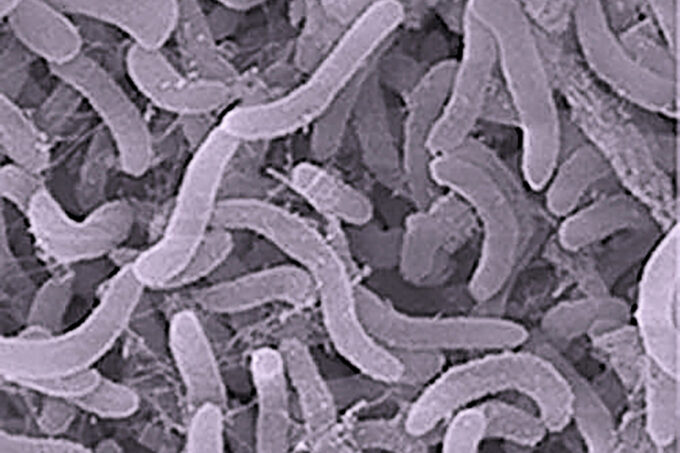
This image shows SAR11, the most common type of bacteria in the ocean. In the study by Zakem et al., SAR11 was found to be the most abundant group, especially near the ocean’s surface. The model used in the study assumes that SAR11 feeds on easily digestible organic material. Image credit: NOAA
In the vast, blue expanse of Earth’s oceans, trillions of microscopic organisms quietly shape the planet’s climate. These marine microbes—bacteria and archaea—play a central role in the ocean’s carbon cycle, influencing how much carbon is stored in the deep sea versus released into the atmosphere. Yet, despite their importance, these tiny life forms have long eluded accurate representation in global climate models due to their staggering diversity and complexity.
Now, a new study offers a breakthrough. By combining cutting-edge genetic data with sophisticated ocean ecosystem simulations, researchers have developed a simplified, yet powerful framework to understand and organize marine microbial diversity and its impact on the global carbon cycle.
The study, titled “Functional biogeography of marine microbial heterotrophs,” appears in Science. It is the result of a multi-institutional collaboration involving Emily J. Zakem and Liang Xu (Carnegie Science), Jesse McNichol (St Francis Xavier University, Nova Scotia), J.L. Weissman (Stony Brook University), Yubin Raut and Stephanie Dutkiewicz (MIT), Elisa R. Halewood, and Craig A. Carlson (UC Santa Barbara), and Jed A. Fuhrman, and Naomi M. Levine (USC.)
A New Lens on Microbial Diversity
“Microbes are the engines of the ocean’s biogeochemical cycles,” says lead author Emily Zakem, a former PhD student of MIT’s Professor Mick Follows and subsequent Simons Postdoctoral Fellow, now a Principal Investigator at the Department of Global Ecology at Carnegie Science. “But their diversity is so vast that it’s been a major challenge to include them meaningfully in climate models.”
To tackle this, the researchers turned to a treasure trove of global genetic data collected over more than two decades of oceanographic expeditions. These data, which capture the genetic fingerprints of microbial communities across the Pacific, Atlantic, and Indian Oceans, were compared to the Darwin ecosystem model—a detailed computer simulation developed at MIT that mimics the interactions of marine life and biogeochemical processes.
Rather than trying to model each microbial species individually, the team grouped the modeled microbes based on two key traits: the type of organic carbon they consume (ranging from easily digestible to chemically complex) and their growth strategy (fast-growing “copiotrophs” versus slow, resource-efficient “oligotrophs”).
Remarkably, this two-dimensional framework was sufficient to explain the large-scale distribution patterns of 21 major microbial groups aggregated from the sequencing data across the global ocean.
Surprising Insights from the Deep
Among the study’s most intriguing findings was the identification of a group of deep-sea microbes dubbed “slow copiotrophs.” These organisms grow slowly, perhaps because they are adept at breaking down tougher forms of organic carbon compounds that are more resistant to degradation and can persist in the ocean for longer periods. This insight provides new information about how much organic carbon sinks or is transported into the deep ocean versus remaining near the surface or returning to the atmosphere.
“These microbes are like the slow but steady recyclers of the deep ocean,” explains co-author Yubin Raut, a postdoc in Mick Follow’s group at MIT. “They’re not fast, but they’re efficient, and they play a crucial role in processing carbon that would otherwise accumulate.”
Microbial Ecology as a Climate Lever
The study further shows that these ecological dynamics are not just descriptive—they are mechanistic levers that influence carbon storage in the ocean. Fast-growing microbes dominate the consumption of easily degradable carbon near the surface, preventing it from accumulating. However, harder-to-degrade carbon tends to build up, mainly because the microbes that could consume it are suppressed by predators.
When these predators are removed in the computer simulation, the slow-growing microbes thrive, and surface dissolved organic carbon storage drops significantly. This means that even small changes in the ocean’s microbial community, due to warming, nutrient shifts, or other environmental changes, could have large impacts on how much dissolved organic carbon the ocean stores.
“Microbial ecology—specifically the balance of growth and mortality—can significantly influence long-term organic carbon storage,” says co-author Stephanie Dutkiewicz, a Senior Research Scientist in MIT’s Department of Earth, Atmospheric and Planetary Science affiliated with the MIT Center for Sustainability Science and Strategy. “That’s one of the major insights here: ocean carbon storage isn’t just about physics and chemistry, but also about who is present in the microbial community and how they interact.”
This finding challenges the traditional treatment of microbes in climate models, which has often been simplified. The study provides a mechanistically grounded reason to include microbial diversity and dynamics in future climate predictions, especially under scenarios of global change.
A Collaborative Effort
This research was supported in part by the Simons Collaboration on Computational Biogeochemical Modeling of Marine Ecosystems (CBIOMES), an initiative that aims to advance understanding of ocean ecosystems through interdisciplinary science. Two researchers, the lead author Emily Zakem, and JL Weissman, were funded through Simons Foundation Postdoctoral Fellowships in Marine Microbial Ecology, while others were supported through the Simons Foundation-funded BIOS-SCOPE program.
The study exemplifies the power of collaboration across institutions and disciplines, from new novel molecular datasets and oceanography to computational modeling and climate science.
“Ultimately, understanding these invisible organisms is key to predicting how the ocean will respond to a warming world,” says Yubin. “They may be microscopic, but their impact on the planet is anything but small.”