What do the rocks say? lab edition
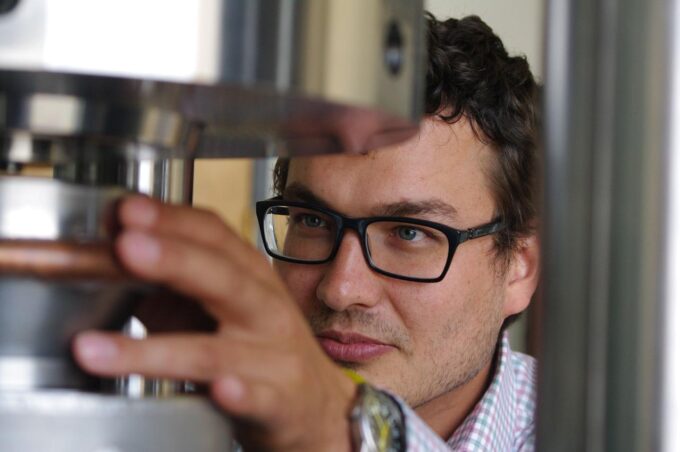
Matěj Peč loads a sample into the deformation apparatus where it will be subject to pressure and temperature conditions occurring deep in the Earth. Image Credit: Helen Hill
Deep inside the Earth, the rocks are singing. The intense heat and crushing pressure below the Earth’s surface causes rocks to deform – to crack, flow, fracture, and melt. In the process, they emit acoustic waves, which can propagate through the Earth as a series of small compressions and decompressions. Some of these waves reach the surface where geophysicists can pick up on these vibrations in the form of seismic waves and use them to learn about the internal dynamics of the Earth. However, low-pitched sounds can travel over much longer distances through the Earth than higher-pitched sounds. So, waves propagating through the Earth are more attenuated the further away from source we are, losing information about small scale processes occurring at the source.
Within the Earth’s crust, it’s not “anything goes” – instead, it’s “everything flows” … eventually. Even what we think of as solid rock will shift and flow over geologic timescales; drifting tectonic plates are a case in point. Rock flow can happen so slowly it can be hard for humans to appreciate, but it still affects life on the planet’s surface in the form of volcanic eruptions and earthquakes.
All deformation is associated with microscopic defects in the rocks. The interactions and collective motion of these defects make deformation possible and ultimately determine whether an earthquake will occur or a volcano will erupt.
To learn more about how rocks deform, researchers recreate Earth’s internal conditions, sometimes as deep as 100 kilometers, within the lab. Scientists subject small rock samples to high temperatures and pressures, and then listen as the rocks respond. The high frequencies generated by the samples are out of reach of the human ear by an order of magnitude, but by using special ultrasound sensors, observation becomes possible. In essence: under enough pressure, you still may not be able to get blood from a stone, but you can get a rock to hint at the stresses it’s feeling and why it can’t take it anymore.
Picking up good vibrations
In MIT’s Experimental Rock Deformation lab, which is led by Victor P. Starr Career Development Chair Matěj Peč, a hydraulic press takes center stage. The press itself is roughly the same height and width of a person. Inside a heavy-duty frame rests a set of central pistons and a gleaming drum-shaped pressure chamber. At the very bottom is another smaller chamber that holds the sample: a tiny column of rock one centimeter long and thinner than a chopstick. By slowly and inexorably pressing down on the rock sample and running electric current around it, the machine gradually builds up the temperature and pressure, until they are comparable to conditions miles below the Earth’s surface.
Some other equipment helps to control these experiments. Amid a controlled chaos of tubes and pipes and electrical wiring, there is a set of pumps to regulate pressure, a heating panel to regulate temperature, a bank of computers to record experimental data, and a water-cooling system to keep the whole collection from going up in flames. And, of course, there are the ultrasound sensors.
“Sensors are everything in this universe,” says Hamed O. Ghaffari, a research scientist in Peč’s group, who designs and builds them for these rock-squeezing experiments. He says that the sensors rely on piezoelectricity: they take a mechanical signal, like the physical pressure you would feel from a handshake or a slap, and convert it into an electrical current, or vice versa.
Certain kinds of crystal are inherently capable of making this conversion, so the sensors used in rock-squeezing experiments are hollow metal needles filled with tiny pieces of these crystals and connected to electric wiring. The sensors are then positioned at different points above and below the tiny rock sample in the hydraulic press waiting for a wave to hit them so that they can record it.
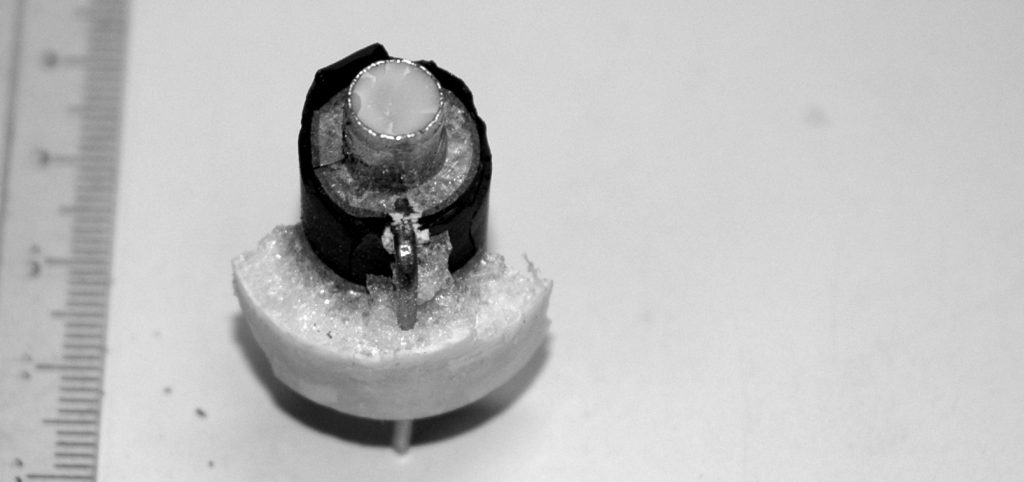
The big squeeze
The rocks geophysicists study are made up of minerals (like quartz, for example, or calcite), which have a crystalline lattice structure. Atoms are arranged in a regular, repeating pattern, lending the rock its strength and rigidity. If all the atoms were perfectly arranged, the rocks would be many orders of magnitude stronger and the Earth would look quite different. However, in the real world, the internal structure of rocks is full of small flaws: a few missing atoms here, a misaligned section there.
These little imperfections are called defects, which will ultimately determine whether the rocks will fracture or flow inside the Earth. Imagine that you are missing one atom in an otherwise perfect lattice, making a point defect. Now you can move all the other atoms around in the crystal just like you can re-park all cars in a parking lot with one empty space — diffusion can take place and can slowly change the shape of the rock. Maybe you have an extra plane of atoms squeezed into the crystal. The termination of this plane will be a line called a dislocation that can move through the crystal and accommodate deformation. At last, imagine that the bonds along a plane in the crystal are separated and you get a defect plane that is a crack. The types of defects that dominate within the crystal depend on the external conditions during their formation.
When the rock sample is squeezed, things start changing. Under enough physical stress, those flawed points begin to give way. Tiny cracks and fractures form or dislocations sweep through the crystals, all emitting high-frequency acoustic waves, each with its own signature sound. The sensors pick up these ultrasound vibrations, and the electrical patterns provide researchers with a hint of how rocks respond to changing conditions within the Earth’s interior.
Surface connections
Rock-squeezing experiments like this help to tell researchers more about the stress limits for a particular type of rock. The answers to this question can have implications for us surface-dwellers. For example, extracting fluids like drinking water and fossil fuels from the Earth – or conversely, injecting substances like wastewater, excess carbon dioxide, or the various fluids used in fracking – introduces change and stress to the surrounding rocks. If those rocks shift and fracture enough in response, it can induce earthquakes that otherwise would not have occurred. Understanding more about how rocks deform could help avoid unintentionally setting off potentially destructive events.
Additionally, it could enable geophysicists to better predict naturally-occurring earthquakes, too. “I can tell you that Los Angeles will certainly be hit by a really big earthquake in the next thousand years,” says Peč. “I’m sure I’ll be right, but of course the usability of this prediction is essentially zero.”
Predicting earthquakes, Peč says, is even harder than the notoriously tricky job of predicting the weather. “With earthquakes, you cannot see anything because it’s underground.” In contrast, atmospheric behaviors can be directly observed using satellites. It’s also relatively easy to study a given weather event because weather patterns occur with a higher frequency. Earthquakes are a far more elusive target for study, because in a given location they recur naturally only on a scale of hundreds to thousands of years.
Fortunately, the lab-based technique of using ultrasound sensors to “listen” in on rocks gives researchers a way to keep learning more about the Earth’s inner workings on their own schedule.
Alice McBride contributed to this piece.